We are focused on "Quantum Nanophotonics" that involves creating and
studying on-chip quantum devices (shown below) and circuits that exploit the first quantum
particle-- the photon-- for information sensing, transfer, and processing utilizing the rules
of quantum mechanics. It is a foundational subset of the general field of "Quantum Information".
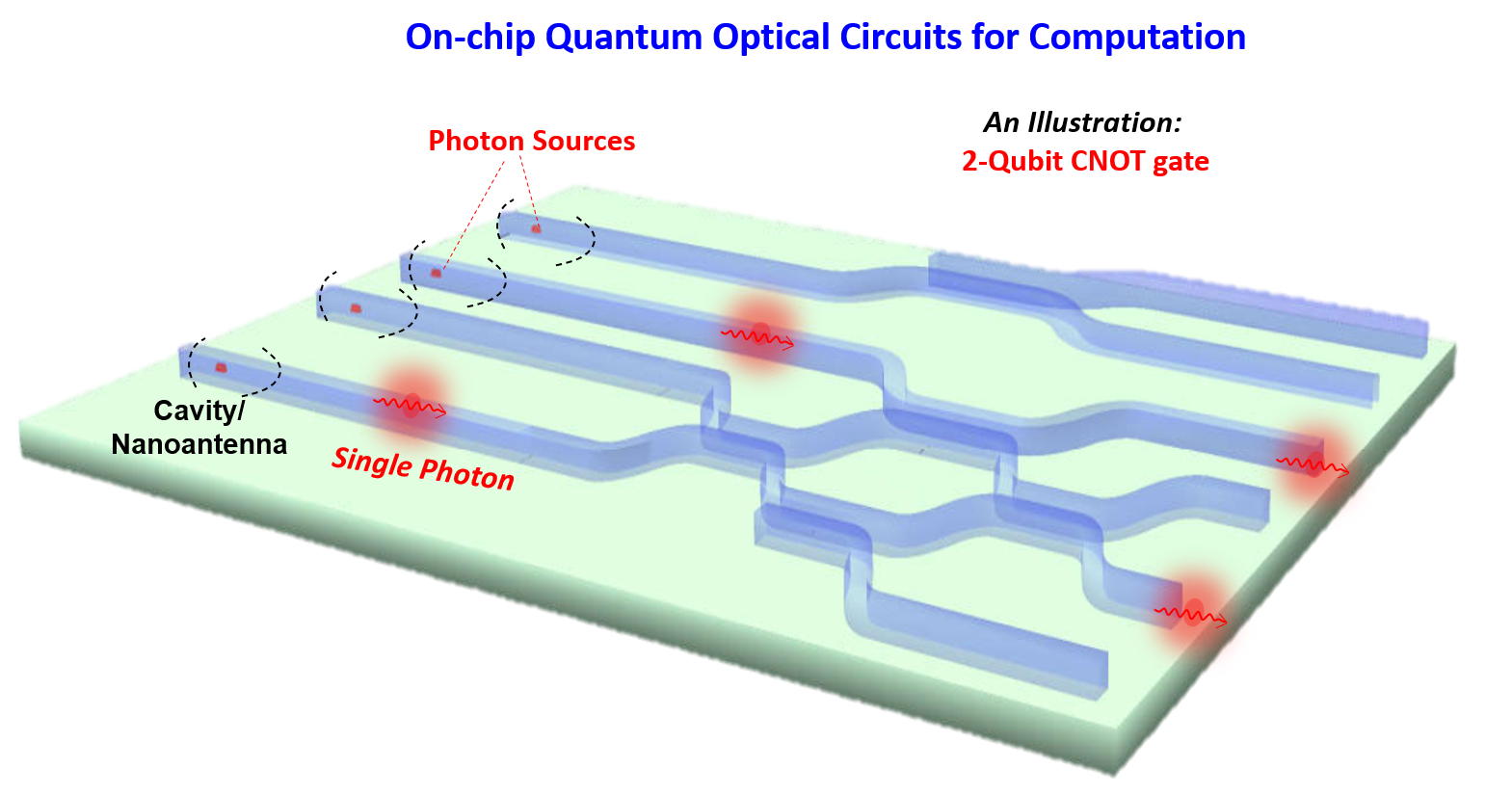
We are using single photons as the quantum particle and create on-chip quantum nanophotonic systems
(figure above) that exploit the rules of quantum mechanics for information sensing, imaging,
communication, and processing beyond what laws of classical physics allow. Targeted areas of
applications range from quantum imaging (i.e. spatial resolution determined by the Heisenberg
uncertainty principle), metrology, quantum repeaters for long-distance secure communication,
and quantum computing. Creating such systems that utilize as few as "single" photon to do the
work requires following the laws of quantum mechanics for designing the systems and their
fabrication requires building blocks such as (1) photon sources generating single photons in
a spatially ordered arrangement and (2) co-designed light manipulating units to control photons
on-chip. All these components are to be fabricated at the nanoscale (typically from 10nm to 200nm)
demanding parallel and well-coordinated efforts in the growth of appropriate combinations of
materials with atomic-level control, their structural and chemical characterization, fabricating
individual as well as interconnected devices (i.e. circuits) and studying their appropriate
optical and electronic properties.
Our work on this involves two major fronts:
(1) Developing the needed ordered on-chip integrable single photon source array utilizing a unique new class of mesa-top single photon sources
(2) Developing the system level design of light manipulating units based circuitry to interconnect single photon source for circuits and device)
Single Photon Source Arrays:
Why do we care about Single Photons?
A single photon can act as qubit--a carrier for quantum information.
The qubit may be encoded in the photon's polarization, number, phase, etc.
Manipulation of single photons and their interference/ entanglement can result in Quantum Communication,
Quantum Metrology, and Quantum Computation for Quantum Information Science.
What is so special about a Single Photon Source?
Single photon sources emit only one photon at a time of only one color (/frequency).
|